Chemical reactions proceed via a mechanism – one or more steps in which electrons are transferred between molecules (‘intermolecular’), or within a molecule (‘intramolecular’). Each of these steps involve an activation energy to occur. This means that the constituent components of the step must collide – or come very close to one another – with sufficient energy to overcome some prohibitive barrier to reaction. Only the step with the highest activation energy barrier in a reaction determines the rate of the reaction – chemists call this the ‘Rate Determining Step‘ (RDS).
Catalysts speed up chemical reactions by providing an alternate route by which the RDS can occur, which has a lower activation energy barrier. Heterogenous catalysts are of a different phase to the reaction they are speeding up. In the gas phase reaction between carbon monoxide (CO) and oxygen (O2) to form carbon dioxide (CO2), a solid ruthenium surface acts as a heterogenous catalyst, speeding up the bond formation process in the RDS. We have long thought that this was by virtue of holding the component parts of the reaction in close proximity, so they are more likely to collide and form products, but we had no way to prove this assertion. The interim state between bonds being formally made or broken is known as the transition state of a chemical reaction. It was previously thought that this was an unobservable state, as it is such a delicate balance of competing electrostatic forces. The frequent analogy is rolling a ball up a hill. The vast majority of the time you will either roll it with either enough energy, and the ball will pass over the hill, or with insufficient energy, and the ball with roll back down towards you. It is very difficult to roll the ball with precisely enough energy for it to stay sat atop the hill in perfect balance. This is a much more tangible analogy of a chemical transition state. It was once thought that we can only ever realistically detect intermediates – when the bonds have been formally created or broken (to run with the analogy from classical mechanics, we can only observe the ball at the next plateau along the mountain range of hills).
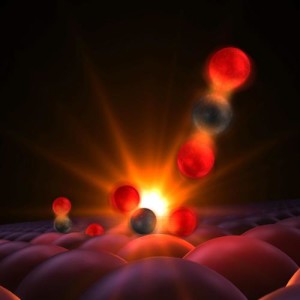
Carbon Monoxide Reacts with Oxygen on the Surface of a Ruthenium Catalyst (http://phys.org/news/2015-02-scientists-glimpse-chemical-bond-born.html)
Scientists at the SLAC National Accelerator Laboratory (operated by Stanford University) have – for the first time – been able to create a ‘flick-book’-style movie of a chemical reaction unfolding. Using femtosecond X-Ray pulses, they were able to ‘illuminate’ the atoms and molecules on the catalyst surface sufficiently quickly to observe the individual bond forming steps (think of a strobe light at a nightclub). First the oxygen molecules are activated – the diatomic molecule splits and individual oxygen atoms are adsorbed (stuck) onto the catalyst surface. Then the carbon monoxide molecules are adsorbed onto the catalyst – this time without the molecule being split. After a trillionth of a second, the two components vibrate and move around enough to collide and the observed transition state was formed – the exceedingly short timescale of these steps accounts for why the pulses needed to be so very short in duration. The carbon of CO forms a ‘translational bond’ to the oxygen atom. Surprisingly, only a very small fraction of these translational bonds exist for long enough for the newly formed carbon dioxide to separate itself from the catalyst and escape.
Why do we care? Well – this is the very same reaction that goes on inside the catalytic converters of cars, where CO is ‘neutralised’ to CO2 before it escapes the exhaust. CO needs to be neutralised as it binds irreversibly to the iron complexes in haemoglobin, which prevents it from reversibly transporting oxygen around the body. A better understanding of how the ruthenium catalyst is working can direct scientists to developing better-tuned catalysts for this process. The group intends to look at many of the other key surface-catalysed industrial reactions, which is likely to spark similar new development for these processes too.
Formerly we could only hypothesise (to a reasonable degree of certainty) the precise order and degree to which bonds were broken and formed on a catalyst surface. Now, we can prove it. This will undoubtedly have immense value in the field of chemical catalysis. For many years we have known that certain metallic complexes catalyse specific reactions, and have attempted to develop theories as to why they do. This is a giant step forward to a world where we can rigorously test these hypotheses, giving synthetic and computational chemists a much more targeted approach to designing the next generation of more efficient compounds.
References
1. http://phys.org/news/2015-02-scientists-glimpse-chemical-bond-born.html (An interesting popular science article)
2. http://www.sciencemag.org/content/early/2015/02/11/science.1261747 (The original scientific paper)